Fungus Eating Flowers: Orchids, Climate Change, and the Nature of Evolution
By: Christian Elliott
A shorter version of this story ran in Sierra Magazine on December 4, 2022.
Dennis Whigham closes the car door, straightens his blue baseball cap and squints into the woodland before him. A maze of planks crisscrosses a forest floor covered with cables and hoses. Little yellow flags wave in the breeze and lights on white metal boxes tied to trees blink on and off. Motors hum as little robot lids open and close, taking methane measurements. PVC pipes support wide nets of leaves above the ground. He smiles.
“Now all I have to do is figure out where the hell we put the seed packets,” he says, stroking his white beard.
This is the Smithsonian Environmental Research Center’s high-tech experimental forest in coastal Maryland. Twice a year, scientists flood it with 80,000 gallons of saltwater to study the effects of hurricanes and sea level rise on forests. It’s a massive, expensive undertaking called TEMPEST. But Whigham’s goal here is different. He, like many other Smithsonian scientists, has hitched a ride on TEMPEST’s infrastructure – International Space Station-style – to do his own experiment. He wants to know how sea level rise will affect Maryland’s native orchids. He’s arrived with his longtime colleague Melissa McCormick to locate and dig up orchid seeds they planted throughout the forest a year ago to see if any have sprouted. It’s not an easy task, but two heads are better than one.

As she walks carefully through the dead leaves, trying not to step on hoses, McCormick points out a small green leaf, speckled with purple.
“Tipularia!” she says triumphantly, bending over to inspect it more closely.
A line of small orchid plants follows a rotting log for a half a dozen feet beyond the leaf she spotted. She flips one over to reveal its brighter purple underside. Tipularia discolor, or the Cranefly Orchid, is not the sort of orchid you find in grocery stores or florists’ shops. One of nine species on the Smithsonian’s campus (and one of 54 native to Maryland), in another few years it will send up a delicate flower stalk covered with small, brownish maroon flowers. It only sprouts on decomposing wood. And like half of all North American orchids, it’s incredibly rare.
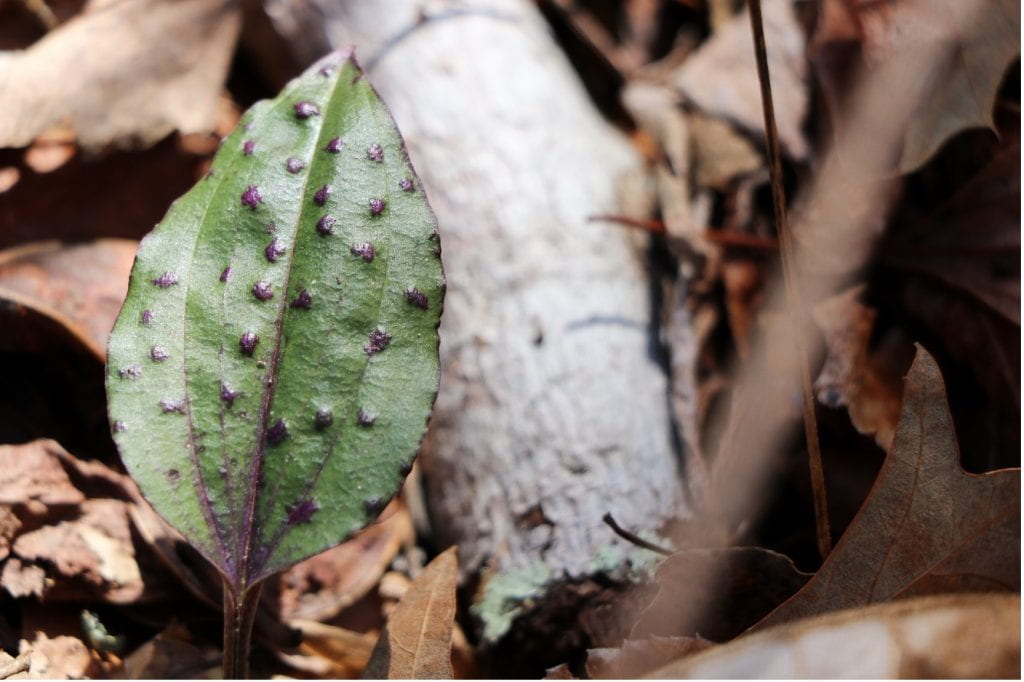
Nearby, Whigham kneels in the leaves, pocketknife in hand. He digs in the soil, uprooting a worm McCormick identifies, with a biologist’s eye, as an invasive species – almost all earthworms here are non-native. Then, up comes a tiny set of squares covered in a layer of woodchips – teabag-like seed packets Whigham designed with a Danish graduate student decades ago to study orchid germination. Now, they represent the international standard method to study wild orchids. He snips off a line of little squares, leaving the rest in the ground for the next four years of this study. Peering into the packet, it’s impossible to see anything besides the soil clinging to the outside.
Orchid seeds are tiny – the size of a single speck of dust. Each plant produces millions. Charles Darwin was fascinated with them. How could a plant that produces so many seeds be so rare? He calculated that if an orchid’s seeds all grew into plants, then the great grandchildren of a single orchid would “cover the earth in one continuous green carpet.” Scientists have identified about 25,000 species of orchid worldwide, more than any other plant family. They represent 10% of all plant species on Earth. They come in a nearly endless variety of shapes and colors. With their diversity and tendency to cross-pollinate, the plants fueled Darwin’s theory of natural selection. Yet about half worldwide are endangered or threatened.
Today’s orchid scientists, like Whigham who has studied the plants at the Smithsonian since 1977, are just as fascinated by orchids. But now, a revolution in genetic sequencing is allowing them to finally answer Darwin’s big questions. What makes something one species and not another?
Popular since collectors first obsessed over it in Victorian England, the charismatic orchid now commands millions of dollars in research. Far from just pretty faces, orchids are allowing scientists to ask fundamental questions about how plants interact with other species in their environment and about the very nature of ecology and how we classify organisms.
Welcome to the Plant Molecular Ecology Lab
Melissa McCormick strides past orchid posters and origami orchids and test tubes of orchid seeds and jars of orchids reaching toward the light of grow lamps. In the Smithsonian’s plant molecular ecology lab at the research center’s main campus in Edgewater, Maryland it’s orchids all the way down. She yanks open a drawer and pulls a plastic baggie out of the darkness. Opening it, she pours a dozen sealed petri dishes out onto the lab table. Each one is filled with a brownish white slime. Barely visible in the slime aretiny white sprouts. She holds one at eye level, pushing her thick rimless glasses up her nose.
“Isn’t that amazing,” she says. “I haven’t looked at these in two months.” Two months – that’s pretty fast for an orchid to sprout. How slow are orchids? The green plants growing on the shelves took eight months to grow just a few inches high. In a few years, they might flower. McCormick points at the sprouts in the petri dishes.
“Those are leaves,” she says. “They’re just white because they’ve been in that dark drawer. If I have them out here for any length of time they’ll green up. But this is fast. Most species, you’re looking at a couple of white dots after two months.”
The slime below the seedlings is fungus McCormick took from the ground where she got the seeds. Some of the petri dishes have it, some don’t. In the ones without, the sprouts are tiny – barely visible. The ones with it are thriving – all growing little white leaves.

Most terrestrial plants form relationships with fungi in the soil—they’re called mycorrhizal fungi for their role in a plant’s root system, or rhizosphere. Usually, the fungus attaches to the plant’s roots, providing essential soil nutrients like nitrogen and phosphorus. In exchange, the plant shares extra sugars it produces through photosynthesis with its chlorophyl-deficient underground friend. Teaming up with fungi helped plants first adapt to living on land when they migrated out of nutrient-rich seas.
“Orchids don’t do that,” McCormick says with a laugh. “They basically parasitize their fungi.” They eat them alive. That’s completely unheard of in the plant community.
Orchid seeds are so tiny they carry no food for a growing plant. To sprout, the seeds rely fully on fungi to provide them with nutrients. That’s how the petri dish sprouts can grow translucent white, non-photosynthetic leaves. No other plant fully relies on consuming fungi to survive.
The fungus forms tight coils within the orchid embryo’s cells (the orchid controls where it grows using a fungicide). Then, the orchid slices open the fungus and absorbs its nutrients, bit by bit. White, nugget-like orchid babies, called protocorms, can then live underground for years, feeding on their fungi and waiting until conditions are just right to emerge above the surface and start doing the work of growing themselves.
But not just any fungi will do for a growing orchid – most species rely on a single very specific species to sprout. By producing a lot of seeds, orchid parents try to guarantee some land where the fungus they need is already living. Some orchids then spend their entire lives digesting their fungal friends, without ever growing leaves to photosynthesize themselves – though most are mixotrophic, eating fungi and photosynthesizing. What’s more, scientists have recently found some orchids require different species of fungus to sprout, live in their protocorm stage, and then live aboveground as adult plants.
“We have the largest living collection of orchid mycorrhizal fungi,” McCormick says proudly. Setting the petri dishes aside, she opens up a cabinet lined with more than 1,200 slime-filled test tubes – each a different fungus species.

“Identifying what these fungi are is really important for orchid conservation,” she says. “Even if you’ve got an orchid plant or you’ve got a bunch of seeds, you still can’t grow them if you don’t have the right fungus. If it’s not there, they’re not going to survive.”
The vast majority of the fungi in McCormick’s cabinet don’t belong to any known species. Since they don’t form a fruiting structure in the lab, which is required to register an official description of a new species, it’s impossible to ID them visually – even under a microscope. In science parlance, they’re “morphologically depauperate,” McCormick says.
“Which is to say, lacking any features that allow you to tell them apart.” She laughs. “Think, a smear of jelly on wood that may or may not become totally transparent when it’s dry. They’re pretty unimpressive looking as fungi go.”
Despite McCormick’s research, orchid mycorrhizal fungi remain a massive question mark – why do they let orchids eat them? What else are they doing in the soil besides associating with orchids? How big are fungus individuals? In a lima bean-sized sample of soil, there could be up to 150 species of fungus engaging in all-out warfare among one another. It’s the subject of serious – sometimes heated – debate among the small global community of orchid scientists.
Figuring Out Fungi
McCormick joined the Smithsonian Environmental Research Center in 1999, responding to a flier on a wall at an ecology conference in Baltimore advertising a postdoc position. After years spent studying grasses, she had some flowering plant envy, she admits. She wanted something that would make a sexier photo on a poster presentation. Whigham was looking for a molecular biologist to help him answer some big questions about orchid-fungi interactions using genetics. McCormick quickly filled half his lab space with genetic sequencing equipment.
At the time, Tom Bruns, the “godfather of mycological genomics” at Berkeley, was starting to develop methods to identify tree mycorrhizal fungi using DNA sequencing. Now, the genetic revolution had reached orchids.
“Fungal identification before this was based on looking at this thing under a microscope, figuring out what kinds of angles its hyphae were branching off at, what kind of model structures does it make. And it turns out that it’s darn near impossible to tell these fungi apart,” McCormick says. “They had microscopes in Darwin’s day, but they sure didn’t have DNA sequencing.”

The process is fairly straightforward. First, McCormick snips off a piece of orchid root and sterilizes it. Then, under a microscope in a fume hood, she carefully pops the fungus balls out of the root piece, washes it again, and grows the fungus on agar plates in the lab. She sequences the fungus genome’s DNA and uses quantitative real-time PCR to figure out how much was in the plant root to start with.
Soon, she’ll be doing this work at a massive scale at the research center – digging into the U.S. Forest Service’s nationwide archive of soil samples, which the agency collects on 5-year intervals. She plans to use DNA primers for all the fungi species she’s identified genetically so far to look at soil samples from 10,000 Forest Service plots to figure out where fungi live and how they vary across ecosystems.
“We’re still at the point of trying to see who all is there,” she says. “We still know very little about what these fungi are doing in the soil, other than associating with orchids.”
In some studies, under laboratory conditions, scientists have detected trace carbon moving from an orchid to its fungus, suggesting some orchids may have more mutualistic relationships with their fungi, like almost all other plants do. McCormick doesn’t buy it.
“I am of the school that thinks orchids always parasitize their fungi,” she says. She recently disproved a mutualism believer’s theory that in times of drought, orchid roots provide a refuge for moisture-loving fungi. It turns out when it gets dry, orchids actually step up their digestion of the fungi, closing off their stomates and ceasing photosynthesis to avoid losing moisture.
The other lingering question is whether the fungi really care. It could be that fungus individuals are so huge underground, that the orchid parasitizing their fungi is “like a mosquito biting us,” McCormick says.

“My thought is that it’s just the fungus foraging for food, and it happens to grow inside the orchid roots,” she says. “It just spreads out, happens upon an orchid and gets eaten a little, but that’s not enough of a selective pressure for it to evolve different behavior.”
“They’re not eating buckets of fungi, they’re just taking a little snack here and there out of it,” Whigham agrees. “I think by sort of staying small and almost inconspicuous, orchids have been able to make this work.”
But how big are fungus individuals? Since they’re underground in a shifting mixture of hundreds of other fungi and bacteria that make up the soil microbiome, that turns out to be a very difficult question to answer directly. By DNA fingerprinting the fungus found in orchid roots in a given area, McCormick can find the limits of a fungus patch through the orchids. If the next orchid over’s fungus has a slightly different genetic profile, it’s growing in a different fungus individual. Thus far, McCromick believes the fungi organism is about a meter in size. But like all things orchids, this answer raises more questions.
“How do you define an individual when you have something that can spread, and you can chop it in half?” McCormick asks. “They’re genetically identical. Are they now two individuals?”
Livin’ on a Prayer: Showtime for Pollinators
Custom-built waterproof motion-sensing camera boxes bounce in the trunk as Melissa McCormick guides the car down the gravel road to the Smithsonian field site. “Livin’ on a Prayer” blasts over the stereo – appropriate, as any orchid needs a bit of luck to make it in the forest. Prayers don’t hurt. The mood in the car is jovial. It’s late April, any orchid scientists’ favorite time of year – the time when orchids start to bloom.
McCormick hops out and pops the truck, carefully lifting two camera boxes, battery packs, and the PVC pipe frames that will support them on the forest floor. It’s time to work on the other half of the lab’s research on orchids – pollinators. She hands one of the cameras to Monica Marcelli, a researcher from George Mason University assisting on the project.
Whigham’s already here, wandering out of the forest in his usual baseball cap. McCormick ribs him about his oversized fishing vest.

“I just needed lots of pockets to put things in,” he says, defensively.
“Now all we need to do is make it through the woods without losing any of these pieces,” McCormick says. A small PVC component clatters to the gravel in response.
Not only do orchids rely on specific fungi during specific stages of their lives to sprout from seed and grow, adult plants also require pollination from very specific insects to produce seeds in the first place. How specific?
Take the Drakaea glyptodon, or “king in his carriage” tropical orchid for example. Its fuzzy purple flower has evolved to mimic the shape and smell of a female thynnine wasp, attracting and tricking male wasps into attempting to mate with it. When the wasp lands, a hinge in the flower moves and bonks the wasp into the stigma, covering him in pollen.
McCormick describes it this way: “The pollinator comes in, gets tricked, goes ‘Well, dammit, I’m not doing that again,’ and flies off somewhere far away. At which point he gets desperate and gets fooled again. So, your pollen goes a long way and crosses with very genetically distinct individuals. It’s a mechanism for promoting outcrossing.”
Climate change is proving a problem for that long-developed evolutionary plan. Orchids are very long-lived, slow-growing organisms. The insects they’ve evolved to attract are not. They operate, evolutionarily, on very different timescales. As seasons change and the Earth warms, orchids and the pollinators they depend on could start missing each other, as insects emerge earlier and orchids bloom the same time of year they always have.
Only half of North American orchid pollinators are known, and most of those have been identified by scientists in only one location. Of course, pollinators worldwide are in decline. McCormick and Marcelli hope to learn what pollinators the orchids that grow on the Smithsonian’s campus here rely on.
Other members of the plant molecular ecology lab – technicians, postdocs and interns – stream into the forest one by one at the rumor of blooming orchids. Together, they traipse through the woods, pants tucked into socks to keep the Lyme-bearing ticks off, the way botanists do – eyes to the ground, scanning for plants. They pass one of Whigham’s old cranefly orchid plots, in which he’s censused and measured every individual plant for more than 30 years.
Then, McCormick spots her target – a Cypripedium acaule, a pink lady slipper orchid. Four inches above the ground, a stack of tiny ballet slipper-shaped blooms wave gently in the breeze. Kneeling down, she calls over the others. Cell phones clad in custom orchid-themed cases crowd around like orchid paparazzi to photograph the season’s earliest bloomer. Then it’s time for work.
What follows is an hour of orchid IT as McCormick and Marcelli set up the two motion detecting cameras – one with night vision and one without – next to the little orchid movie star. McCormick takes particular joy in yanking out a couple of invasive species blocking the shot. Marcelli reads off IP addresses as McCormick sets up the local Wi-Fi network and writes down the password, orchid1!. Gotta remember those special characters. Then it’s just a matter of focusing the video.

A camera-shy bumble bee buzzes by just as the cameras come online, circling the orchid then hiding underneath some dead leaves.
“We have a pollinator already,” Marcelli says, waving at the bee. “Come here, come here!” It does not.
“How are you planning to separate the bugs who want to pollinate the orchid from the ones that want to make it big on the silver screen,” an intern jokes.
Marcelli answers, earnestly, with the list of potential bumble bee pollinators and how an entomologist will help her identify them. This orchid has 20 potential bumblebee pollinators. With any luck, she may find out which ones actually pollinate the orchids here. Or, they could end up with a lot of footage of a lonely orchid swaying in the wind.
Family Orchidae and the End of Ecology
Ida Hartvig sits in front of the computer in her office in the Smithsonian’s main research building, phylogenetic charts and bioinformatics data on her monitor. She’s back on campus from her home in Denmark for another week to finish up analyzing data she collected as a postdoc before the pandemic. Ten days into the data, she takes a walk in the woods to clear her head.
“I’m a little fried,” she says.
In the forest behind the lab, she points out a little Goodyera oblongifolia plant, the rattlesnake plantain orchid, stroking its striking white veined leaf, which looks like the way cracks spiderweb across glass.
A botanist in her natural habitat, she points out spring beauties, the intricate design of a sweetgum seedpod, and a centipede that may be a worm – that’s not her department.

Years ago, Hartvig was a strait-laced botanist studying species delimitation of timber trees. Several Smithsonian orchidologists spent their PhD years the same way – doing taxonomic revisions, using genetics and morphology to refine the established boundaries between species, categorizing and recategorizing plants as one subgenera or another. Also like many of her fellow orchidologists, an orchid mystery drew her away from trees and into a more complex world. She visited a forest in Denmark where managers had cleared trees around one surviving orchid individual– the only one of its species left in the country. In 10 years, there were 1,000 individuals there, as the fungi they needed to survive exploded with so much tree root matter to decompose.
“I’d been so focused on plants my entire life,” she says. “It is what I do; I am a botanist. But now, that really opened my eyes to the importance of fungi and of all the other microorganisms.” She began to think of the forest in relation – maintained through interactions between species. For her, orchids broke down the longstanding boundaries between scientific specializations that love to consider species in isolation. The next radical step, Hartvig says, is hologenetics – studying the entire soil and plant microbiome (since many microbes live within the tissue of plants) together to understand the genome of an individual orchid. That’s when she returns to Denmark.
“We’re just beginning to learn that microorganisms, really the microbiome of the dirt, has an outsized impact on plants,” she says.
But for now, at the Smithsonian, her work is raising another big question – one that first germinated in Darwin’s orchid-filled mind over a century ago and has rippled repeatedly through the fields of biology and ecology in recent years – what makes a species?
Hartvig is studying two species of fringed bog orchid, the white Platanthera blephariglottis and yellow Platanthera ciliaris. Like many orchid species, these two lack clear morphological mechanisms that prevent cross-pollination, and so they readily hybridize.
“Our theory was that the adaptation to associate with a specific fungus might be involved in speciation patterns,” Hartvig says. Could fungi be driving another species – orchids – to differentiate and evolve to occupy different ecological niches? It’s a chance to see evolution in progress.
Painstakingly, she hand-pollinated orchids and germinated their hybrid seeds with fungi in the lab. So far, she’s found a very clear pattern in fungal use – the orange hybrid children of blephariglottis and cilaris seem to be able to use the mycorrhizal fungi of either parent. She found a clear genetic component in determining what microorganisms orchids associate with. That discovery has major implications for orchid conservation. Should we prioritize hybrids or “pure” populations?
“If your conservation focus is on the natural processes of evolution and conserving genes, but maybe not the individual combinations of those genes the make up a given species, then these mixed populations are super important,” McCormick says.
Hybridization could become more common as orchids slowly but surely migrate as the climate changes, seeking lower, wetter areas. Some species move faster than others and could overtake them, promoting cross-pollination.
“Hybridization is really important for evolution of new species,” McCormick says, “And for the generation of the kind of genetic diversity that allows species to tolerate things like changing environmental conditions.
But there were also a few key exceptions in Hartvig’s findings – plants that appeared to be hybrids in the field that couldn’t use both parents’ fungi. Harvtig sequenced them and was surprised to find a “mosaic of genotypes” where she expected a clear phylogenetic chart.
She calls them “cryptic hybrids,” impossible to identify as one species or another morphologically in the field. And they call into question biology’s intense focus on categorizing and classifying – a practice that dates back to Carl Linnaeus, the father of binomial nomenclature and modern taxonomy. Meticulous classification and subcategorization was also the focus of Hartvig’s PhD research, before her eyes opened to the bigger picture.
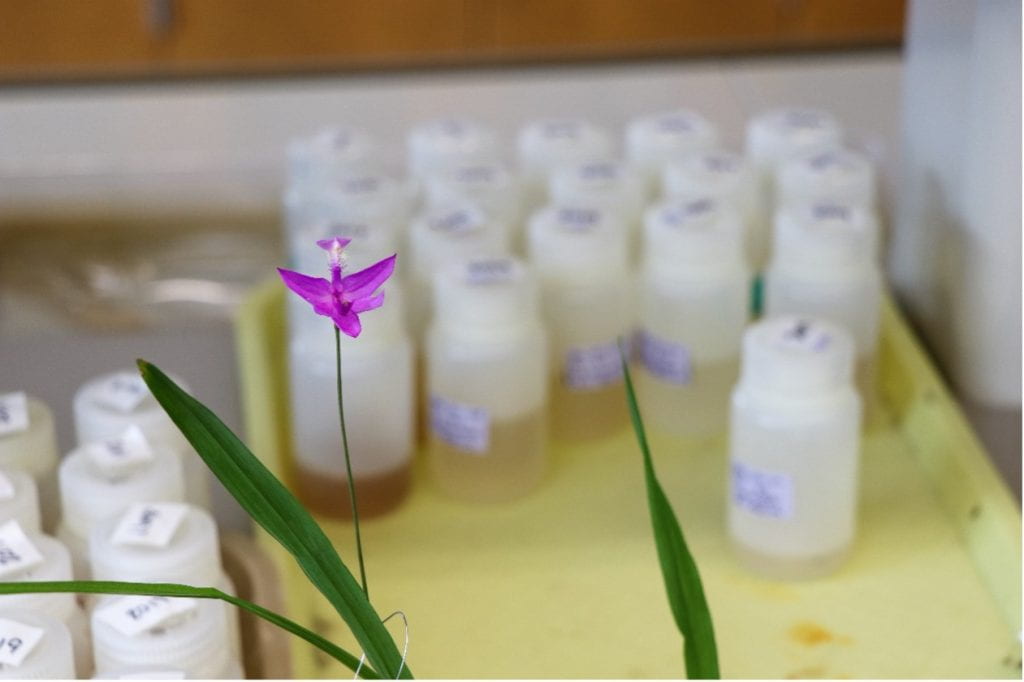
“There are so many orchid amateur botanists out there that just love to look at orchids and all the variation, and they love to describe a new variety and argue what is one species and what is another,” Hartvig says. “But our preliminary data shows there may be in some geographic areas, just a lot of hybridization such that in that area we cannot effectively distinguish between one or another species.”
It’s a good reminder ecology isn’t static, she says. Evolution is an ongoing process, and orchidologists are looking at plants at a snapshot in time. Species definitions aren’t as solid as they appear printed in the pages of a biology textbook.
“When you’re in the middle of something happening evolutionarily, you know, the game’s not over,” says Whigham.
“I’ve learned to be comfortable with that over time,” Hartvig says. “I can now say, ‘OK, this is an individual of this purple complex, and I can’t say completely whether it’s one species or another or a hybrid if I see it in the field.’ But still, a lot of people will try very hard to define it by morphology.”
McCormick agrees. “At this point it’s pretty clear species definitions are kind of going by the wayside,” she says. “There just aren’t clear breaks all the time; it’s often more of a continuum. At some level, where you define a species, the exact position of that line can be a bit arbitrary, like someone just decided, ‘Alright, this is it. We’re gonna make the cut off here.’”
The more you look at orchids, the more those lines blur and tangle, like the fungi and orchid roots hidden just underground.
The Plants Have More Stories to Tell
“The truth is, there could be just as many interesting stories with less appealing plants,” Hartvig says. “Orchids, they’re pretty to look at so they get a lot of attention. There are other plant species which have these complex relationships and interesting evolutionary histories.” Orchids are one of the few plants not subject to “plant blindness” in research funding and literature that disproportionately goes toward what she calls “brown furry things.” The human beings who allocate funding are biased towards our fellow mammals. That’s a problem in a changing world in which we desperately need to understand how the soil microbiome affects the plants we need to grow to survive.
For now, this small group of orchidologists in coastal Maryland, and small research groups like them across the world, will keep digging in the soil, keep growing slime in test tubes, keep watching protocorms turn to flowers over the long years, keep an eye on the cranefly orchid plots, and keep contributing to our understanding of how plants work together with other species and use each other to survive and adapt.
“We certainly don’t have many of the answers yet,” Whigham says. “What all this shows is there’s no standard anything. Each species is on its own schedule and has its own interactions with other things.”
Someday he hopes to have seeds and fungus samples from every one of North America’s 200 species of native wild orchid, to capture and preserve the sheer genetic richness of the family Orchidae.
But it’s only been 40 years. Not much time to the orchids, or to the people who’ve dedicated their lives to better understanding these strange, diverse, slow-growing plants.
Christian Elliott is a science journalist and audio producer based in Illinois. He’s reported science and environmental feature stories for National Geographic, Science, Scientific American, Undark, Sierra, and more. He’s produced podcasts and radio stories for WBEZ, Deutsche Welle and NASA. He received his master’s in science journalism from Northwestern University’s Medill School in Chicago.
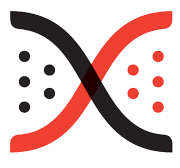